Researchers use optical and scanning electron microscopy to describe the phenomenon of self-ejecting crystals from heated, nanotextured superhydrophobic materials
Water is a vital part of our every day lives. In developed countries, water also plays a critical role in cooling thermoelectric power plants. Evaporation is part of the cooling process and can result in mineral precipitation on equipment surfaces, also called mineral fouling. Mineral fouling can have devastating and costly effects on equipment.
Dr. Kripa K. Varanasi is a Professor of Mechanical Engineering at the Massachusetts Institute of Technology (USA). The focus of his work is in understanding the physico-chemical phenomena at interfaces and developing novel materials, devices and products that can dramatically enhance performance in energy, water, agriculture, transportation, medical and consumer devices.
Dr. Varanasi, along with his former graduate student, Dr. Samantha McBride (now a presidential post-doctoral fellow at Princeton University, USA), recently published a paper which describes how so called “crystal critters” can self-eject from heated, nanotextured superhydrophobic materials during evaporation of saline water drops. The crystal critter effect has potential applications for improving sustainability in spray cooling heat exchange.
Crystal critter
Tell us about your laboratory at the Massachusetts Institute of Technology (MIT)
The Varanasi Lab focuses on how micro and nanoscale processes at the interfacial level can be manipulated to tackle big industrial problems. For example, fouling (the accumulation of contaminants in pipes, reactors and/or membranes) is a critical global challenge affecting the energy, desalination and chemical industries. Monetary losses due to fouling account for 0.25% of the GDP of industrialized countries and fouling is currently a limiting factor in the efficiency, material lifetimes and overall sustainability of many processes. Although the impacts are large, the onset of fouling is an inherently interfacial process that must be understood on a microscopic level in order to be prevented.
Our approach is to develop a rigorous thermodynamic framework to identify the true bottlenecks and then figure out efficient kinetic pathways to impart the solution.
By understanding the phenomena, it becomes possible to reduce even the largest problems down to a few nondimensional parameters and thus collapse complex processes into manageable formulas and phase diagrams.
These can then be used to design new processes, new products and zero-tradeoff solutions. These strategies can be applied to any problem where interfacial effects lead to critical bottlenecks. We work on problems ranging from aircraft anti-icing, drug delivery, efficient application of pesticides to crops and water harvesting.
What is the crystal critter phenomenon?
In this publication, we were interested in how the length-scale of superhydrophobic surfaces influences crystal fouling from evaporating drops. Superhydrophobic surfaces are created by a combination of hydrophobic chemistry with a surface topology on the micro- or nano- length scale. Many superhydrophobic surfaces utilize micro-scale topologies with characteristic length scales of about 5 to 30 microns. However, a number of previous investigations have found that such superhydrophobic surfaces fail to prevent crystal fouling and that hydrophobic materials often out-perform superhydrophobic ones.
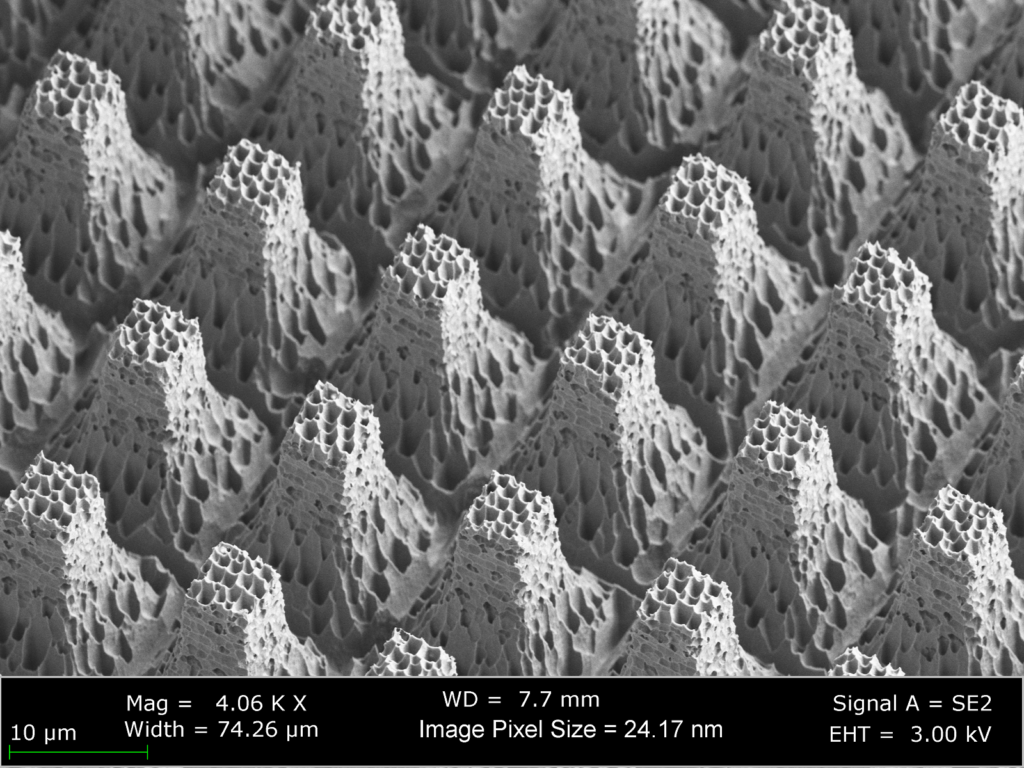
A side-view image acquired using scanning electron microscopy capturing details of one of the superhydrophobic surfaces used in this study. The superhydrophobic texture has multiple length scales and is composed of micro-scale post features and nano-scale nanograss features.
We hypothesized that this failure was a function of the topology length scale and that nano-scale confinement effects would prevent crystal intrusion and therefore eliminate crystal fouling.
In addition to confirming this hypothesis, our experiments on superhydrophobic nanotextured surfaces (characteristic length scale <1 micron) also revealed that these materials enable a dynamic self-ejection of crystal structures during evaporation. This self-ejection is a rather unusual consequence of the combined effects of crystallization, evaporative flux and nanoscale confinement of growing crystal forms. The lifelike motion of the crystal structures during growth as well as their final morphology composed of a crystalline “body” balanced on a set of “legs” led us to name these structures “Crystal Critters.”
How did you use microscopy in this publication?
We used both optical microscopy and scanning electron microscopy in this publication.
During experiments of critter growth, we used a ZEISS light microscope to record the dynamics. These videos allowed us to identify local regions of fluid impingement into the nanoscale texture and demonstrate that both the position and size of those regions correlated with the position and diameter of critter legs. This was critical in understanding where and how critter legs first began to form, which was key to understanding the self-ejection phenomena.
A drop of salt water is deposited on a nanotextured superhydrophobic surface heated to 80 degrees C. Crystals form as the drop evaporates until a point at which the water de-wets from the substrate and crystal legs begin to grow. Evaporation leads to leg growth until the water is completely gone.
We used a ZEISS scanning electron microscope (SEM) to characterize the length-scale of our nanotextured material and to capture high resolution images showing the material topography. Following growth and self-ejection of the crystal structures, we imaged locations at which critter legs had previously grown to search for any crystals or salt residue at those locations. We were able to find regions of extremely thin layers of salt residue. These regions were not readily visible using the secondary electron detector; however, by switching to the inlens detector, we were able to create a stark contrast between areas with and without salt. This enabled us to collect data regarding the typical size of legs on the microscale.
Scanning electron microscopy (SEM) was also used to demonstrate that the failure of microscopic superhydrophobic surfaces is caused by crystal growth and intrusion into the texture while crystals did not grow within the nano-scale texture.
The final application of scanning electron microscopy in this work was to capture details of the tips of the salt legs of the crystal critters. These images were captured using the secondary electron detector at a low voltage to prevent charging effects of the sodium chloride crystals. The microscopic crystalline legs were exceptionally fragile and difficult to image, but we were able to preserve them long enough for imaging by gently blowing the crystal critters from the substrates onto pieces of scotch tape.
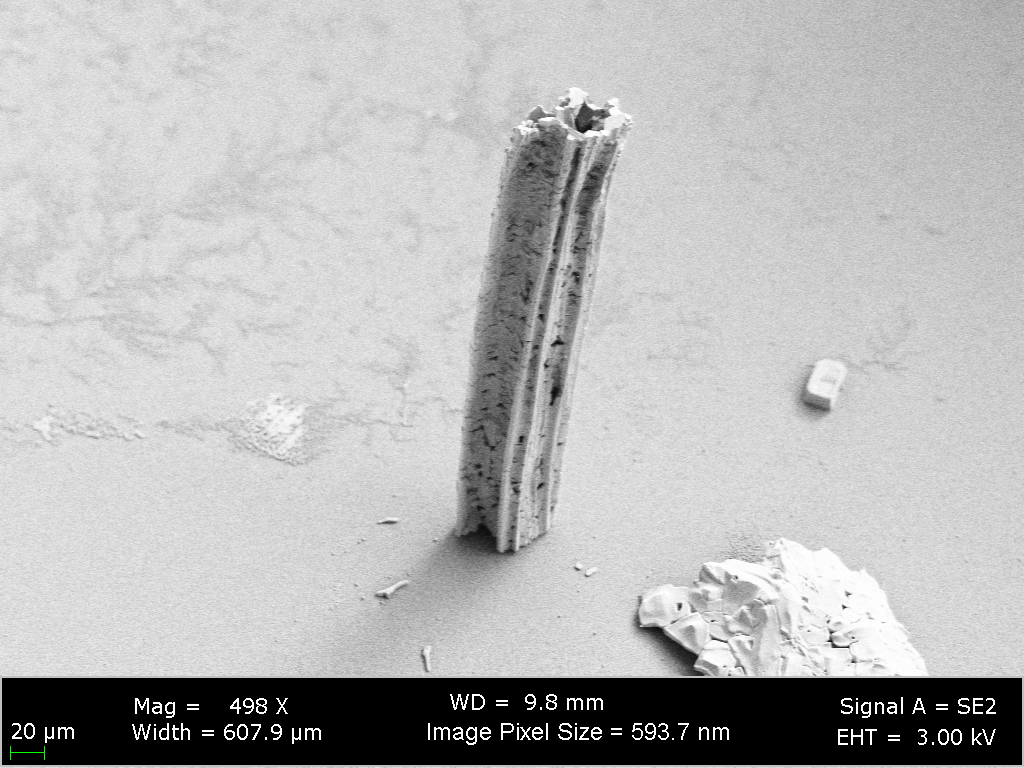
A crystal critter leg, showing that these structures are hollow tubes. Imaged by scanning electron microscopy (SEM).
Where will this work go next?
Next, our team plans to study how the crystal critter effect can be used in practical applications such as in heat exchange. In order to understand this, we are testing the limits of what types of surfaces can be used to induce the effect and what influence water chemistry has on the ejection phenomena. These results may also be useful for creating salt-resistant coatings for marine and coastal environments.
ENTREPRENEURSHIP
Dr. Varanasi is passionate about entrepreneurship and has co-founded multiple companies such as LiquiGlide, DropWise, and Infinite Cooling for translating the technologies from his lab to market.